In 2024, the Nobel Prize in Chemistry was awarded to three scientists who revolutionized the field of protein research, leveraging artificial intelligence (AI) to predict and design protein structures.
Demis Hassabis and John Jumper of Google DeepMind, along with David Baker of the University of Washington, were recognized for their groundbreaking contributions to protein structure prediction and computational protein design.
This award not only marks a major scientific achievement but also highlights the growing role of AI in advancing fundamental research and tackling long-standing biological problems.
The work of these scientists opens new possibilities in medicine, materials science, and biotechnology, offering potential solutions to some of humanity’s most pressing challenges.
Demis Hassabis and John Jumper: AlphaFold and the AI Revolution
Demis Hassabis, the founder of DeepMind, and John Jumper, who led the development of AlphaFold, have redefined the approach to protein structure prediction. Proteins, essential molecules that drive almost every biological function, consist of long chains of amino acids that fold into complex three-dimensional shapes.
Read : John Hopfield and Geoffrey Hinton Awarded Nobel Prize in Physics
The folding patterns of these chains determine how proteins function and interact with other molecules, making the accurate prediction of their structure a critical problem in biochemistry. For decades, scientists struggled with what became known as the “protein folding problem,” a task that seemed almost impossible due to the sheer complexity of protein structures and their chemical sequences.
Read : Global Academic Achievements: The Top 10 Universities in the World
In 2020, Hassabis and Jumper made a major breakthrough with the development of AlphaFold 2, an AI model capable of predicting protein structures with remarkable accuracy based solely on their amino acid sequences. This innovation was transformative for biology, solving a puzzle that had eluded researchers for over fifty years.
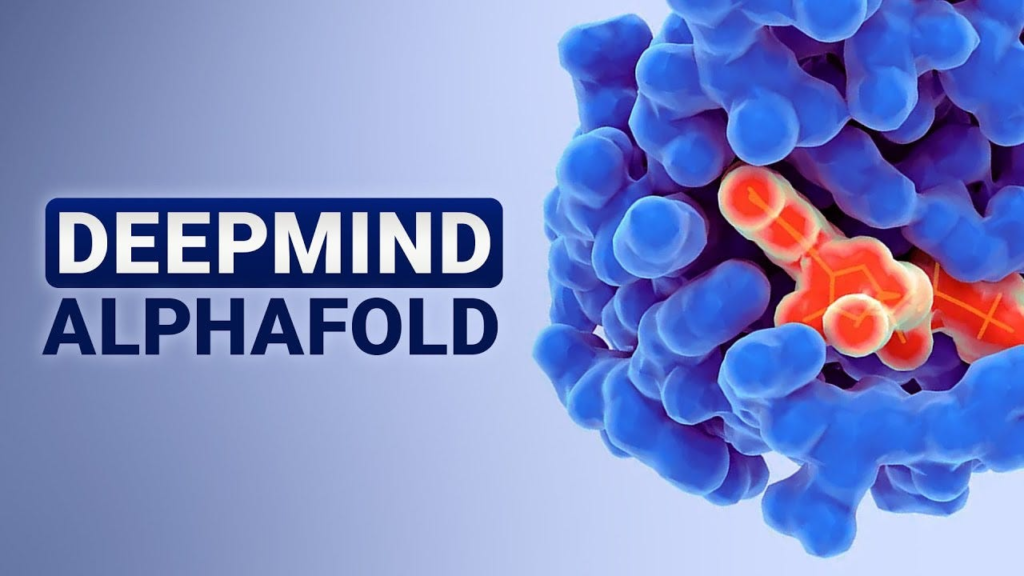
AlphaFold 2’s deep learning algorithms allowed it to predict the 3D structures of proteins by analyzing vast amounts of data, identifying patterns that were previously invisible to human researchers.
The implications of this achievement were enormous. For the first time, scientists could accurately model the structure of virtually any protein, opening up new avenues for drug development, vaccine design, and understanding biological mechanisms at the molecular level.
Since its release, AlphaFold has become a widely used tool in scientific research. More than two million people from 190 countries have applied AlphaFold in a variety of fields, ranging from understanding antibiotic resistance to creating enzymes that can break down plastic.
The model has predicted the structure of nearly all the 200 million proteins known to science, a feat that would have been unthinkable without AI. Researchers across the globe now rely on AlphaFold to accelerate their work, and its influence is felt in everything from fundamental research to practical applications in medicine and environmental science.
Hassabis and Jumper’s accomplishment has also highlighted the dual nature of AI. While the technology holds immense potential for accelerating scientific discovery, it also comes with risks. As Hassabis himself noted, AI is a “dual-purpose technology” that can be used for both good and harm.
The challenge for society is to harness AI’s positive applications while mitigating its potential negative impacts. Nevertheless, AlphaFold stands as a testament to the power of AI to solve complex problems and improve human life.
David Baker: Designing New Proteins for a New Era
While Hassabis and Jumper focused on predicting existing protein structures, David Baker of the University of Washington took a different approach: designing entirely new proteins that do not exist in nature.
Baker’s work has opened up an exciting new frontier in biochemistry, with the potential to create custom proteins for a wide range of applications, including vaccines, nanomaterials, and molecular sensors.
His research represents a fundamental shift in how scientists think about proteins—not just as biological molecules to be studied, but as building blocks that can be engineered for specific purposes.
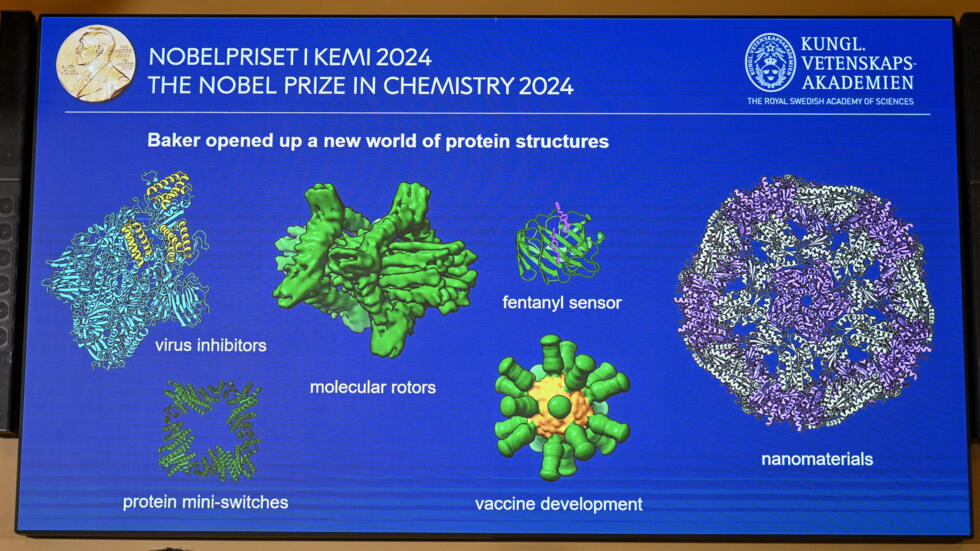
Baker’s journey began over two decades ago, driven by the dream of creating novel proteins that could address some of humanity’s most pressing challenges.
In 2003, his team succeeded in designing the first synthetic proteins, a breakthrough that laid the foundation for what would become a major field of research. By harnessing the power of computational tools, Baker’s team was able to design proteins with precise structures and functions, something that nature had never produced. These synthetic proteins have a wide range of potential uses.
For example, they can be engineered to act as vaccines, triggering immune responses against specific pathogens, or as nanomaterials with unique properties that can be used in manufacturing and technology.
One of Baker’s most significant contributions has been in the field of vaccine design. During the COVID-19 pandemic, his research group applied their protein design techniques to develop novel vaccines.
These efforts have demonstrated the real-world impact of computational protein design, showing how artificial proteins can be created to solve urgent medical problems. Beyond vaccines, Baker’s work has also led to the development of proteins that can serve as sensors, capable of detecting everything from environmental toxins to biomarkers for diseases.
In addition, his team has created proteins that could revolutionize materials science, offering new possibilities for constructing nanoscale devices and materials with unprecedented precision.
Baker’s vision of designing new proteins to solve 21st-century challenges is becoming a reality, thanks to advances in both computing power and scientific understanding. His work complements the achievements of Hassabis and Jumper by demonstrating the creative potential of computational biology.
While AlphaFold solves the problem of predicting protein structures, Baker’s research takes the next step: creating new proteins that can be tailored to meet specific needs. Together, these advances represent a paradigm shift in how scientists approach the study and manipulation of proteins.
The Broader Impact of AI and Computational Biology
The 2024 Nobel Prize in Chemistry not only recognizes the individual achievements of Hassabis, Jumper, and Baker but also underscores the transformative potential of AI and computational biology.
Proteins are central to all biological processes, acting as enzymes, hormones, and structural components of cells. The ability to predict their structures and design new ones has far-reaching implications for fields as diverse as medicine, agriculture, and environmental science.
One of the most immediate applications of protein structure prediction is in drug development. Many diseases, including cancer, Alzheimer’s, and infectious diseases, are caused by the malfunction of specific proteins.
By understanding the 3D structure of these proteins, scientists can design drugs that precisely target them, potentially leading to more effective treatments with fewer side effects.
AlphaFold has already been used to predict the structures of proteins involved in various diseases, speeding up the drug discovery process and enabling researchers to explore new therapeutic approaches.
In agriculture, protein design could lead to the development of crops that are more resistant to pests and diseases, reducing the need for chemical pesticides and improving food security.
Proteins designed to interact with specific environmental factors could also be used to engineer crops that are better suited to withstand climate change, such as drought-resistant plants or those capable of thriving in nutrient-poor soils.
The environmental applications of protein design are equally exciting. Enzymes, a type of protein, can be engineered to break down pollutants or even convert waste materials into useful products.
For example, scientists have already created enzymes that can decompose plastic, offering a potential solution to one of the world’s most pressing environmental problems. In the future, synthetic proteins could be used to clean up oil spills, remove heavy metals from water, or capture carbon dioxide from the atmosphere.
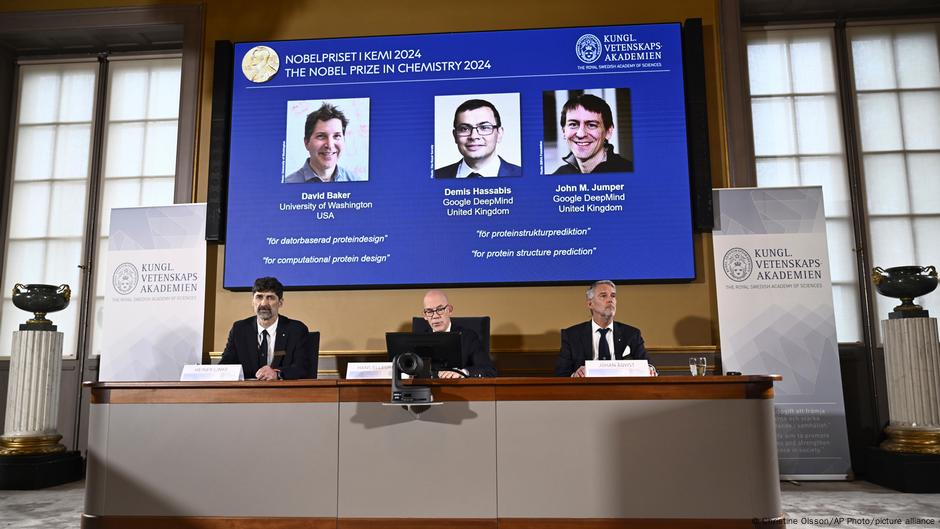
Despite these promising developments, the use of AI in biology also raises ethical and societal questions. As Hassabis pointed out, AI has the potential to be used for harmful purposes, and it is essential for researchers, policymakers, and society as a whole to carefully consider the implications of this technology.
The ability to design proteins could, in theory, be misused to create harmful biological agents or to manipulate biological systems in unpredictable ways. As AI and protein design continue to advance, it will be crucial to establish safeguards and ethical guidelines to ensure that these technologies are used for the benefit of humanity.
The 2024 Nobel Prize in Chemistry awarded to Demis Hassabis, John Jumper, and David Baker marks a significant milestone in the fields of AI, computational biology, and protein research. Their work has not only solved long-standing scientific problems but also opened up new possibilities for medical treatments, environmental solutions, and materials science.
By harnessing the power of AI, they have demonstrated how technology can be used to accelerate discovery and address some of the most pressing challenges facing humanity. As we look to the future, the work of these scientists will undoubtedly continue to inspire new innovations and applications, shaping the way we approach biology and technology for years to come.
let’s enjoy few years on earth with peace and happiness….✍🏼🙏